One of the ubiquitous examples of the Hückel rule is cyclooctatetraene dianion (COT2-). This annulene has, presumably, 10 π electrons and therefore should be aromatic, satisfying the 4n+2 rule. Therefore, the molecule should be planar, right? Well, an article by Dominikowska and Palusiak call into question these assumptions.1
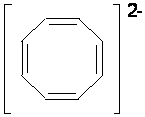
COT2-
First off, with either B3LYP or MP2 and a variety of basis sets, optimization of COT2- starting from the tub-shape of COT itself led to the planar or nearly planar structure most of the time. The exceptions include B3LYP/6-311++G(d,p) and MP2/aug-cc-pVDZ. More interesting is that a number of the MP2 planar structures have one or more imaginary frequency; for example, MP2/6-311G has four imaginary frequencies.
I reoptimized a number of these structures assuming D8h symmetry, and looked for the number of imaginary frequencies. B3LYP/6-311G(d,p) had no imaginary frequencies, but B3LYP/6-31++G(d,p) and B3LYP/6-311++G(d,p) had 2 and 4 imaginary frequencies, respectively. Many of the MP2 optimizations had imaginary frequencies, with MP2/6-311G(d,p) having 3 imaginary frequencies. The optimized structures of COT2- at ωB97X-D/6-311G(d,p) had no imaginary frequencies but with the 6-311++G(d,p) basis set, it had two imaginary frequencies. Interestingly, Truhlar’s M06-2x functional with both 6-311G(d,p) and 6-311++G(d,p) gives no imaginary.
This is reminiscent of the situation with benzene and other arenes, where certain combinations of method and basis set gave multiple imaginary frequencies.2 The ultimate culprit was identified as intramolecular basis set superposition error. Dominikowska and Palusiak discount this explanation here for two reasons. First, multiple imaginary frequencies are seen with the Dunning correlation consistent basis sets – MP2/aug-cc-pVDZ has 7 imaginary frequencies (though my computation at D8h gives only one imaginary frequency), something not observed for benzene. Secondly, they noticed that in the non-planar COT2- optimized structure there are bond paths connecting the hydrogens to non-nuclear attractors situated way outside the molecule. They suggest that the COT2- might really be a Rydberg state, with the extra electrons located outside the molecule. This implies that the π system has only 8 electrons, giving the tub shape. They note that COT2- has a very short lifetime and suggest that it is not an aromatic compound, a larger annulene congener of benzene, at all.
It would be interesting to see what would happen with COT2- correcting for intramolecular basis set superposition error via the method of Asturiol, Duran and Salvador,3 which I described in this post. This correction led to planar benzene having no imaginary frequencies. This type of computation would help assess just what is going on here – is COT2- afflicted with basis set problems or is it a very unusual, non-aromatic system?
References
(1) Dominikowska, J.; Palusiak, M., "Cyclooctatetraene dianion—an artifact?," J. Comput. Chem., 2011, 32, 1441-1448, DOI: 10.1002/jcc.21730
(2) Moran, D.; Simmonett, A. C.; Leach, F. E.; Allen, W. D.; Schleyer, P. v. R.; Schaefer, H. F., III, "Popular Theoretical Methods Predict Benzene and Arenes To Be Nonplanar," J. Am. Chem. Soc., 2006, 128, 9342-9343, DOI: 10.1021/ja0630285
(3) Asturiol, D.; Duran, M.; Salvador, P., "Intramolecular basis set superposition error effects on the planarity of benzene and other aromatic molecules: A solution to the problem," J. Chem. Phys., 2008, 128, 144108, DOI: 10.1063/1.2902974